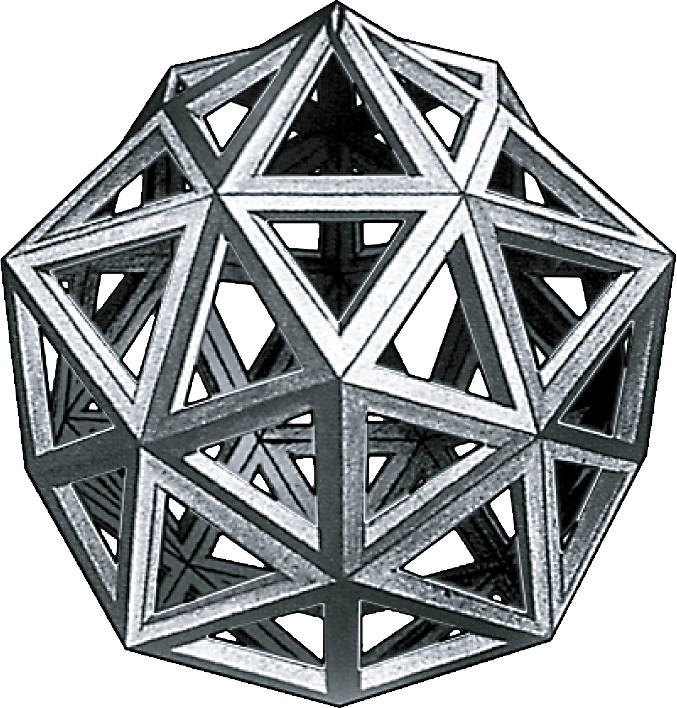
This episode of Manufacturing the Future is brought to you by EOS.
There are many ways to build parts with additive manufacturing technology, but regardless of the additive technology or build strategy, the basic rules of physics are the ultimate constraint, as in other forms of part making. Mechanical, thermal and sometimes chemical properties of materials are critical to consider in part design and build programming in additive manufacturing, and especially so when building with metals.
Heat is a factor in any part making process using metals, but additive manufacturing adds new levels of complexity, as a layered build process. With complex, delicate, or high aspect ratio parts, supporting the structure is a major consideration for dimensionally stable parts. Some new technologies are now available to circumvent some of the classic constraints of part support and dimensional shift due to heat.
Joining Jim Anderton to discuss these new technologies is Davy Orye, Team Manager, Additive Minds Consulting at EOS.
Episode Transcript:
To see any graphics, images, and/or videos to which the transcript may be referring, please watch the above video. The transcript has been edited for clarity.
Jim Anderton: Welcome to Manufacturing the Future. There are many ways to build parts with additive manufacturing technology, but regardless of the additive technology or build strategy, the basic rules of physics are the ultimate constraint, as in other forms of part making. Mechanical, thermal and sometimes chemical properties of materials are critical to consider in part design and build programming in additive manufacturing, and especially so when building with metals. Now, heat is a factor in any part making process, but additive manufacturing adds new levels of complexity as a layered build process.
With complex, delicate or high aspect ratio parts, supporting the structure is a major consideration for dimensionally stable parts. There are new technologies now available to circumvent some of the classic constraints of part support and dimensional shift due to heat. Joining me to discuss this complex issue is Davy Orye, Team Manager Additive Minds Consulting with EOS. Davy has a decade of experience in the additive manufacturing industry and holds master's degrees in both mechanical engineering and management from KU Leuven in Belgium. Davy, welcome to the program.
Davy Orye: Thank you for having me here.
Jim Anderton: Davy, there's so much to talk about this issue and you have a unique layer of expertise, multi-dimensional expertise, in this subject going back to your research days, I understand. But could we define the basic parameters of this problem at this point? What are the difficulties that the part designer have to think about when they think about supporting a structure for an additive build?Davy Orye: Indeed, it's a very complex topic. And even before, let's say, the manufacturing engineer starts, even in the design you already have to think about how am I going to orientate the part? As a result of that, where do I need to have support? And so that brings a lot of complexity to already even before you're printing apart. And then when it comes to support structures, there's also a lot of reasons why you have supports. So the first main reason is you're very locally heating up in DMLS and powder bed fusion. You're very locally heating up your part, your powder, with a laser and that heat has to go away. Typically, if you're printing in the middle and the bulk of your part that goes through your part, through the base plate and so on, it's dissipated really well. But on overhangs where there's only small connections to the rest of your part, you typically add support structures.
Second reason for support is also typically you work with some kind of recoding system, and that recoding system can put a little bit of force on your part and then sometimes the supports are just simply there to make sure that that force doesn't result into a small deformation of your part or bumping of your part. And then lastly, because of what I mentioned before, this local energy input that creates actually quite big terminal gradients into your part, which then in turn results into terminal deformations. And supports can help you either deal with those gradients and lower those gradients or to prevent the deformation which can happen.
Jim Anderton: That's an interesting and complex process with something you mentioned here, which occurred to me, which I'm sure many part designers don't consider, which is that the support of the part is more than just holding the part in the correct orientation during the build. You're saying that there's a heat flux, there's a heat flow that goes through the supports that changes the local heating environment. When you're thinking of as the part distorts during the build or perhaps after the build as it cools, how much does the designer have to consider heat flow through supports or lack of supports?Davy Orye: That's the problem. It's a very complicated topic, this whole heat flux, that even, let's say, the most up-to-date simulation software these days, they cannot even comprehend it or it takes literally days and months to simulate, basically, a complex geometry. So there's only so much as a design engineer and a process engineer you can really understand, and there's only so a human brain can understand. And so therefore, typically, you boil it down to certain design rules, saying, okay, overhangs of 40 degrees in the past typically needed support or certain sizes of parts. So the bigger the size of the part, the more stresses you have in the effort, the more deformations. And so then all of these complex thermodynamics basically are being boiled down into a combination of design rules and then the expertise and experience of application engineers.
Jim Anderton: In other part making technologies, for example, a weldment, fabricating an assembly, or perhaps even in some molding, die casting or injection molding applications to talk about plastic materials, there are strategies you can use to control this deformation. Strategies in the cooling for example or strategies in how long the part is held in the mold or fixtured during the cooling process. In the case of additive, it's sort of a dynamic like a continuum. You have a layer of heat which kind of works its way through the part from beginning to the end. And so does this mean that the designer has to think differently about the way the part cools as well as how it's built?Davy Orye: Indeed. When you're designing, of course, for example, if you think about complex structure, maybe a topology optimized structure, you don't to think about, let's say, the end product. But you have to think about all the intermediate stages when you're building that part, layer by layer. And so things which in the end product are very stiff and wouldn't deform even due to all the thermal stresses or since things are not connected during the build process, you have to take that into account as well when you are determining your orientation in your supporting strategy. So yes, it's quite complicated from that point of view, and that's why still, it's a difficult technology to adapt or adopt where a lot of expertise is then being transferred also through my team, for example, to our customers in order to really help them understand the possibilities, limitations of the technology.
Jim Anderton: Traditionally, if you're perhaps a mechanical engineer and you're working with a different metallic material, things like the thermal properties of the material, modulus, these are sort of handbook solutions. So we know that if we're going to make a part out of mild steel versus Inconel, for example, we can simply consult the database and know how the material properties will change. Is the same process true in additive manufacturing? Is it a matter of I can make something basically out of an aluminum alloy and just translate those parameters directly to, say, a super alloy, or do I have to change the way I designed the part?
Davy Orye: We can probably just about that question talk for hours, but to give you an idea of, let's say, the differences. So if you think, for example, aluminum. Aluminum has a very high heat conductivity. So the energy, even though you put it in there, very locally, very quickly dissipates throughout the rest of your part. So thermal stresses also because of the lower melting temperature, thermal stresses are actually relatively lower in aluminums, at least, let's say, the traditional 3D printed aluminums. If we go to high strength aluminum, that's another topic again. But for example, aluminum solution 10 magnesium, their stresses are definitely not your problem. It's more about heat management.
If we go then to Inconel, for example. There we very quickly get into overheating. We very quickly get into having burned away material and changing even the chemistry of it. And there we really have to manage the heat a lot better. On the complete other side of things here, for example, titanium, where you can put a lot of energy in there and titanium will behave okay. But the stresses and the willingness to deform are really high because you have exactly the opposite behavior of aluminum where you have a higher melting point and a very low heat connectivity. So that energy stays very locally and therefore we have huge, huge amount of stresses in titanium. So if you have a titanium part, especially when they're large, you have to deal with those stresses. And as a designer, you have to know, okay, this will be very difficult for big parts from a stress point of view, where an aluminum, that's absolutely not a problem. And then an Inconel, for example, I have to think about making sure that my overhangs are not burning away and having a good support strategy there.
Jim Anderton: Are residual stresses a factor with advanced part making with this technology? Is a post-processing, a heat treat, something that you see with metal additive?
Davy Orye: Yeah, almost always. And typically, dependent on the material, but typically it's either just a stress release or combination of stress relief and then a further vent heat treatment to get the micro structure you really want. Because typically out of AM compared to other technologies, for example, casting with very fine micro structure, which comes with a lot of benefits because you have that combination of high strength and relatively high ductility. But you can change with a heat treatment where exactly you are on the trade-off between strength and ductility.
Jim Anderton: Additive is an interesting process. I think an example might be, say, an aluminum where if you're used to casting an aluminum part, typically, especially die casting it, you could expect to see, of course, those elongated columnar grain structure near the surface next to the cool surface and more equiaxed grain structure in the middle, if you section these things. But in additive, in this case, you don't have that differential between the core and the outside shell of the part down there. How does that change the way you think about the metallurgy of the part?
Davy Orye: To set expectations, I'm not a super expert on that side. However, I think one challenge, what we do have is, especially when we think also about coppers and so on, is that... And if you think about multi laser systems, so every four or even more lasers all working together on a part, you start to see some kind of gradient. You do start to see some kind of gradients in your part, because what happens at the very start of your build in DMLS, the powder, the chamber, everything is relatively cold and you have a certain process going in there.
But typically, at least, state of the art today is that process kind of remains relatively constant throughout the build. But everything is slowly starting to heat up, especially if you want to be high productive, which obviously we as EOS want to be. And then you start to see certain gradients also in your micro structure because of the heating up of that whole building platform. But it's of course where some of our recent innovations come into play to manage that and keep also the micro structure and therefore mechanical properties and so on much more constant throughout the build.
Jim Anderton: Tell me about that management process because we know that the build process itself is software controlled, and the design process of course is designing the part and designing a strategy for controlling the laser, but it's what can the design engineer, the manufacturing engineer, do about managing the heat or just managing the process on that higher level that you're talking about? Is there something built into the equipment or built into the design software they can use?
Davy Orye: I'll talk about first what we have today and what our customers are today successfully using, and then I'll also talk about the very near future. So today what we have is basically a couple of things. For example, first of all, if vectors and scan pads are becoming very, very short. You have very thin mold applications, basically. These things tend to overheat because you don't have a lot of material to suck away that heat. The laser is moving back and forth really quickly. And so there we have something called a minimum vector time, where basically you wait for a very short amount of time, we're talking here about microseconds, in order to let things cool down a little bit. If you say, "Hey, we don't want to wait," then we have also something called a power reduction factor where based on the length of that vector, we can reduce the power and therefore manage the energy.
The second thing, what I was mentioning is if you go throughout the build and having a heating up, to control that, what we typically do today is, for example, set a minimum layer time. Because especially when layers are very quickly built on top of each other with four lasers, the layers will follow up on each other very quickly and things start to overheat. And you can say, even though the layer takes 10, 20 seconds to build, maybe wait every layer a few additional seconds to let things cool down. However, that's the current stage today, but we don't like that. We don't like to wait because waiting is non-productive time and we want to make sure our customers can use the machine the fullest, and it doesn't make sense if we have four lasers but we have to wait half of the time, then we could just as well have two lasers. And that's where one of our newest innovations comes in, which is called smart fusion.
And smart fusion basically works based on our OT monitoring, like monitoring equipment. OT stands for optimal tomography, and it's basically an easy word to say a thermal camera looking at your whole build plate and at your process. And it captures, basically, the energy of your process. And so it can see which regions of your part, your build, start to overheat. So it knows what the correct heat input should be. It knows basically what the correct color of your melt pool should be. And when things start to overheat, it will recognize that, and then the next layer will adapt your process accordingly. So in other words, if it sees overheating, it will slightly reduce the energy input to compensate for that. And it will, in that same compensate layer, will again look at it and will see if it compensated well and then continue that iteration of cycles in the next layer, which basically allows you to do a much better heat management than waiting, because here we're not losing any time and we have, again, that high productive process which we all love.
Jim Anderton: Davy, is there a trade-off between optimal thermal management and build speed? Everyone of course wants to program to build the part in the shortest time possible.
Davy Orye: So for us, whenever we are developing solutions but it's now for terminal management or for avoiding supports, which we started talking about before. For us, all of these solutions should start with, let's say, the best possible business case and therefore also the most highly productive process. And so the cool thing about our technology is that even though we work based on real data, which is captured while we're building, all of the calculations which need to happen actually happen during the recoding. So in other words, we don't lose any time for these calculations, and our compensation basically is adjusting our laser power and therefore we also don't slow down the process or put specific waiting times in there. So whatever you program as this is the speed I want to build at, the smart fusion software will basically build it at speed and manage the energy input through variations of laser power.
Jim Anderton: We've studiously avoided talking about applications, but there's no way we can talk about an interesting part making technology without discussing applications. Everyone wants to make jet engine hot section blades with this technology. It's sort of the marquee application, and you can do things that are more interesting than, say, investment casting in that application. But we at engineering.com are seeing a lot of excitement about two areas in particular. One is nuclear, small modular reactors, which are some of the hottest energy technology and very topical now in Europe and in the rest of world, of course, with political considerations.
And the other is electric vertical takeoff and landing. The idea of passenger carrying drone technology, multiple rotors, pilot autonomously. Both those have unique requirements. The EVTOL need electric motors that are very light, but of course will require some advanced technologies of cobalt alloys, very interesting things. And of course nuclear, naturally, of course that's a high radiation environment. That has its own challenges at that point. Do you see this type of metal additive, tightly controlled metal additive technology you're talking about being something which is going to be embraced quickly by those two sectors?
Davy Orye: Traditionally, we had the aerospace of course, and space is also definitely still a big market for us, a big booming market at the moment, especially in the US. And the medical where I have been doing a lot of things, you can also see that in my background. There's some hip stands. But I do see indeed, in nuclear particularly... So I'm not involved in everything, of course, at EOS, but in nuclear particularly, I see that there's a lot of interest. There's a lot of interesting projects here at EOS as well. We're also developing some new materials specifically for that industry. For example, around Zirconium, I understood that there is a lot of applications there. Also, Tungsten is one of the strengths of EOS, and there's also quite a bit of applications there. So definitely we see that.
And then also, which I think to some extent is on line with what you were saying is in the e-mobility, as we call it here at EOS. We do see a lot of applications. For example, we're quite front runners in printing copper, although at Formnext I learned that our competition is also starting to catch up there. But we see a lot of copper applications in e-mobility for heat management, also for then the electrical motors and so on. These are definitely growing strategic markets for us.
Jim Anderton: Davy, final question. Looking forward to the future, we hear a lot about generative design, the application of AI to the engineering process, digital twin, model-based systems engineering. When we integrate metal additive manufacturing into that world, into that paradigm, are we going to look at an automated future, do you think, where a designer designs a part basically and the machine says, "This part must be made additively, and I've taken care of it. It's programmed. Parameters are in place. Go." And you press the green button and walk away. Is that the future?
Davy Orye: I hope it is. There's, of course, still some steps to be done there. Let's say, as EOS, we're setting ourselves up for that future. So as EOS, we understand, let's say, additive manufacturing is one of the technologies out of many and you have the best applications if you can combine many manufacturing technologies together where it makes sense. And from a pure software point of view, we make sure that our software can talk to other software, is fully integrable, that you can make your digital twin, that all our sensor data and so on is standardized. So also from a software point of view, really setting ourselves up to be perfectly fit into the factories of the future. Because we understand that that's where things are going and that additive manufacturing plays a very important role in that flexibility or to enable that flexibility. So I'm very excited to see where that all ends up.
Jim Anderton: An exciting future. Davy Orye, EOS, thanks for joining me on the program.
Davy Orye: Thanks for having me once again.
* * *