Gas turbine engines are common in aerospace applications where they provide thrust for jet propulsion. Conceptually simple, the internal combustion engine consists of at least a compressor, a combustion chamber and a turbine. In practice, these components must be carefully designed and manufactured from costly materials to withstand the high temperatures and stresses encountered during operation.
Combustion chambers for gas turbine engines can take more than 25 steps to manufacture
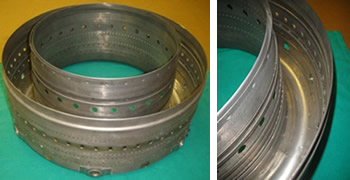
Turbine Engine Combustion Chamber Liner used
on the Beechcraft King Air
Functional efficiency for the combustion chamber relies on its design, its surface roughness, as well as its material and mechanical properties. Usually, the combustion liner tends to be manufactured over a period of time ranging from 5 to 8 months. All in all, it can take more than 25 steps to assemble each chamber out of 6+ single components.
Each part is formed using thin (0.8/1mm thickness) sheets of temperature-resistant alloys sheets that are successively punched, cut to size, hot pressed and hand-brazed or welded together before surface polishing and final inspection. Fuel injection nozzles, swirlers and turbulences-generating features are usually brazed at a later stage. This requires qualified manual labour for manipulation and mounting, setting up rigs and manipulating each sub-component.
Reducing costs and delivery schedules with Selective Laser Melting
Costs and delivery schedules used to be limited by the manufacturing techniques available and the numbers of steps involved. Now, the flexibility of 3D printing makes it possible to create these chambers in a 1-step process. NASA, European Spatial Agency, and GE have already officially built and publicly tested 3D printed high-value components such as rocket fuel injectors. Complete engines are being built by private companies and public research centres using various additive manufacturing techniques ranging from cold spray techniques to direct laser melting while using a range of commercially available metal powders.
Using Selective Laser Melting (SLM) on powder bed, a combustion chamber only requires 45/65hours of build time. In addition, counter intuitive (re)designs to improve combustion efficiency can be more readily built and bench-tested, potentially using customised or exotic powders. With its inherent high-resolution (down to 0.1mm) and its low as-built surface roughness (Ra<7/8um for vertical walls), SLM on a powder bed is well suited for this application, unlike electron beam melting technology with its typical Ra ≥40/50um.
In the case of combustion chambers, SLM is used to test one-off novel designs and to manufacture spares for repair. In addition, SLM on powder bed is also used as a reliable production technique for unconventional or hard-to-manufacture designs, based solely on functional efficiency and unrestricted by conventional manufacturing limitations.
How about post processing of the 3D Printed component?
CAD file of combustion chamber for
1-step machining using AM.
However, if required, even adding post surface treatment such as high quality ultrasonic polishing (final Ra<0.05um) remains a timely and economically viable solution if we consider an additional time estimate of 40/50hours at cost of US$750/1250 per component. Even simulation and modelling times (6months) of a full chamber design can be eliminated in favour of bench-tests. Building a combustion chamber such as the one mentioned earlier using heat resistant nickel alloys powders (US$80/100 per 100Kg) and large SLM production machines, such as EOS M400 (RRP 1.4M€ new) or Concept Xline 1000R (RRP 1.5M€ new), under argon inert atmosphere (US$500/1000 per 8-bottles pack), turns out to be 20 to 30% cheaper than the conventional manufacturing routes.
Selective Laser Melting: an attractive option for aerospace
In the quest to lower aircrafts emissions, save weight and improve engines' fuel combustion, efficiency will play a major role. 3D Printing is at the forefront of aerospace research to achieve this aim. In the case of gas turbine combustion chambers, SLM on powder bed has proven to be an attractive and commercially viable option.
Selective Laser Melting has been shown to reduce delivery schedules by 5 months and save up to 30% in costs. And that's just the fabrication stage. Applying this technology to the design and prototype stages allows for much more rapid iterations of components designs, thereby compounding the benefits of 3D Printing in aerospace applications.